Mission Defiance: Design of a UAS & a Titan Exploration Mission for the Search of Signs of Life
- Setondji V. Nahum
- May 16, 2021
- 15 min read
Updated: Jul 18, 2022
Course: Graduate Capstone
Request a copy of my capstone research paper prior to an interview by sending me an email at snahum29@gmail.com. This is the only option for accessing/reviewing the Acknowledgements, Methodology, Results, Discussion, Conclusion and Recommendation sections of my Graduate Capstone Project.
Acknowledgements
Available Upon Request by contacting me at snahum29@gmail.com prior to an interview.

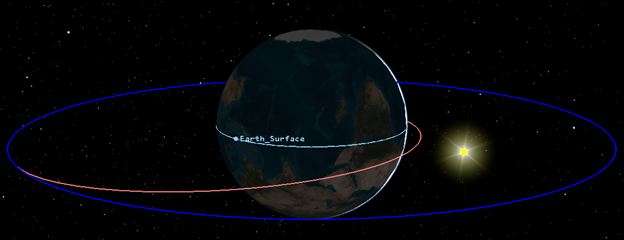
Abstract
The purpose of this research is to design an appropriate UAS and a solar body exploration mission for the search of signs of biological life on Titan. It aims to define tools, processes, and methods for achieving such a mission objective. A UAS is currently the most efficient system for this mission because of its capability for a higher rate of search across Titan’s surface, the use of temperature sensors configured with life search algorithms, the use of synthetic aperture radars (SAR) and advanced digital cameras for high-resolution images and surface mapping, and the superior system and mission safety parameters compared to manned systems which must address human physiological limitations and the threats that the space environment present to humans. This research encompassed Titan and Earth’s characteristics and atmospheric data, aircraft design and space mission planning principles. The research methodology is a proof of concept with three distinct phases. The first phase is a quantitative analysis that defined mission parameters for an Earth to Titan mission. The second phase is a quantitative and a qualitative analysis used to select and define appropriate UAS characteristics and configuration for the Titan exploration mission. The third phase constituted of a definition of a plan and schedule for an observational research in natural settings of Titan based on the defined UAS capabilities and constraints due to the Titan environment. The launch date for Mission Defiance was determined as August 14th, 2021. The spaceflight duration is approximately 3100 days. Reaching Titan was achieved through Hohmann transfers and multiple maneuvers. The Defiance UAS is configured as a foldable fixed wing aircraft with Vertical Take-Off and Landing (VTOL) capabilities to address the need for stable hover flights. A hybrid hydrogen fuel cell and electric engine configuration was selected to maximize system endurance capabilities. The Defiance UAS is equipped with an SAR and temperature sensors built with a life search algorithm to ensure mission success. Signs of biological life search activities on Titan are expected to be completed within 8 Earth Days.
Introduction
The cosmos is a complex system of interacting sub-systems and components mainly constituted of galaxies, stars systems and other cosmic bodies. The milky way is a galaxy that encompasses multiple star systems including our solar system which is composed of the sun and planets such as Earth, Jupiter, and Saturn. Based on recent scientific discoveries, it was determined that the seed of biological life and natural resources on earth emanated from stardust generated by exploding stars (Kwok, 2013). This is important because it supports the theory and idea that there is a strong mathematical probability for the existence of biological life elsewhere in our solar system and the universe. Although significant progress has been made in the field of space exploration by pioneering entities such as the Soviet/Russian Space Exploration Program, the National Aeronautics and Space Administration (NASA) and the European Space Agency (ESA), the cosmos is still to humans mostly an enigma with multiple intriguing puzzles. One of these fascinating puzzles is Titan: a Saturn’s moon that exhibits Earth-like characteristics.
Titan was discovered by the Dutch Astronomer Christiaan Huygens in 1655. It is the largest moon orbiting Saturn and the second largest moon in the solar system. It is characterized by a radius of approximately 2575 km, a gravity of approximately 1.354 m/s2 and an orbital velocity of approximately 5.58 km/s (Coustenis & Taylor, 2008). Titan’s most mesmerizing characteristics include Earth-like features such as an atmosphere, weather patterns, a rocky surface, mountains and volcanoes, dunes, lakes, craters and the presence of liquid methane, liquid water, hydrogen and nitrogen (Coustenis & Taylor, 2008). This makes Titan an ideal place for the search of signs of extra-terrestrial biological life and further knowledge of our solar system. Achieving such an objective requires the design of an unmanned solar body exploration mission due to human limitations and safety factors. In fact, multiple space exploration missions have been completed by unmanned systems including the Soviet Venera missions to Venus, NASA’s Perseverance mission to Mars and the ESA’s upcoming Jupiter Icy Moon Explorer (JUICE) mission.
UAS are integrated sets of multiple sub-systems, components, parts, technological hardware, and software designed for the purpose of flight but not directly piloted by a human. Their advantages include rapid deployability, low development and operational cost, and high system safety parameters (Valavanis & Vachtsevanos, 2015). Although UAS are ideal for the exploration of distant intra-solar planets, solar bodies, and extra-solar planets, they must be adapted to the specific atmospheric and flight conditions on the solar body. A UAS is currently the most efficient system to search for signs of biological life on Titan because of its capability for a higher rate of search across Titan’s surface, the use of temperature sensors built with biological life search algorithms, the use of radars and advanced digital cameras for high-resolution images and surface mapping, and the superior system and mission safety factors ratings compared to manned systems which must address human limitations and the threats that the space environment present to humans (Guastello, 2014).
The research presented in this proposal analyzed titan’s atmospheric data, aerodynamic, astrodynamics, fluid dynamics, propulsion, aircraft airframe, payload, sensors, guidance, vehicle control, navigation, and communication principles, and UAS build requirements to design an appropriate UAS configuration for the search of signs of biological life on Titan. The study used orbital mechanics principles, satellite launch characteristics and requirements, orbit and trajectory determination principles and data to define satellite launch parameters, orbits, and trajectories for the designed UAS. Finally, the research evaluated Titan physical and atmospheric characteristics and the defined capabilities of the designed UAS components such as propulsion system, radar systems and communication systems to define a plan and a schedule of activities for the search of signs of biological life on Titan.
Significance of Study
Biological life on planet Earth is under a constant risk of extinction due to space threats such as solar flux and radiations, earth-bound asteroids and cosmic bodies, and supernovas. For example, solar evolution models confirmed the long-term unsustainability of biological life on planet earth. Within the next three to six billion years which is relatively minimal with respect to the universe timescale, solar flux will generate deadly temperature to earth’s biological life (Schroeder & Smith, 2018). Therefore, space exploration is not a luxury but a necessity that will foster the survival of earth’s biological life. This requires the acquisition of further knowledge about the universe, other planets and cosmic bodies and the development of multiple human habitats across the solar system and the cosmos. Achieving such an objective necessitates the exploration of other solar bodies such as Titan which presents promising characteristics towards the possible existence of biological life within its geological features and or on its surface (Lorenz & Mitton, 2010). With its rocky surface, atmosphere, weather patterns, lakes, dunes and the existence of liquid methane, liquid water, frozen water, nitrogen, and hydrogen, Titan also present fundamental characteristics of a space environment that can be adapted to Earth’s biological species (Coustenis & Taylor, 2008).
This research is significant because it provides tools, processes, and methods for determining whether biological life forms exist on Titan. It also provides tools, processes, and methods for mapping the entire Titan surface and inner-surface water layers, acquiring high resolution pictures of its surface and geological features and further human knowledge about Titan, the solar system, and the cosmos.
Statement of the Problem
The main problem is that temperatures on Titan are extremely low. Titan’s mean surface temperature is reported as -179o C which is significantly lower than the coldest temperature ever recorded on Earth’s surface -89.2 o C in Antarctica (Campbell et al., 2013). This limits the possibility of the existence of earth-like biological species on the surface of Titan but does not negate the possibility of biological life within its geological features or within the surface of the planet. Titan’s extremely low temperatures also add a level of complexity to the design of a UAS because the aircraft’s airframe, structural components, powerplant, radar and communication sub-systems must be capable of functioning within such extreme conditions. The secondary problem is that Titan’s atmospheric composition is different than Earth’s atmospheric composition and therefore the air on Earth is not the fluid within which a designed UAS shall achieve long-duration flights. Aerodynamics, propulsion and especially flight mechanics principles must be adapted to Titan’s physical and atmospheric characteristics such as gravity, fluid density and pressure. As opposed to flying within an atmosphere mainly composed of nitrogen and oxygen, the designed UAS must achieve long-duration horizontal flight and Vertical Take-Off and Landings within an atmosphere mainly composed of nitrogen and methane. The tertiary problem is the determination of optimized orbits and trajectories from Earth to Titan that is free of obstructions and collision risks.
Purpose Statement
The purpose of this proof of concept is to design an appropriate UAS and a solar body exploration mission for the search of signs of biological life on Titan. It provides tools, processes, and methods for determining whether biological life exist on Titan. It also contributes to advancing humans knowledge of Titan, the solar system, and the cosmos.
Research Question and Hypothesis
Despite temperature, atmospheric, gravity, density, viscosity, pressure and meteorological challenges, there is a possibility for the existence of signs of biological life on Titan. Although unmanned aerial systems provide means to mitigate human factors limitations for the purpose of exploring such extra-terrestrial bodies, their design must be adapted to the expected flight conditions on the solar bodies. Moreover, spacecraft launch parameters, orbital trajectory data and navigation parameters must be precisely calculated to avoid collisions with space debris and other solar bodies. Therefore, what are unmanned aerial systems design characteristics and Titan exploration mission parameters for an efficient search of signs of biological life?
Composite materials, fuel cells, solar panels, a hybrid fixed wing airframe with VTOL capabilities, autonomous flight systems, synthetic aperture radars, electro-optical sensors, infra-red sensors, advanced digital cameras, accelerometers, gyroscopes, inertial measurement units and the use of the Deep Space Network (DSN) optimize the design for an efficient Titan-bound unmanned space exploration mission for the purpose of searching for signs of biological life (Atkins et al., 2017). Through program management methods, planning and scheduling techniques and the use of advanced software and mathematical computation tools such as Satellite Tool Kits (STK), Orbital Determination Tool Kits, and MATLAB, mission personnel and flight dynamics engineers compute and determine spacecraft launch parameters, orbital trajectory data, navigation parameters which include trajectory correction maneuvers, and descent parameters (Uhlig, 2015). A pre-defined UAS flight plan and an integrated autonomous flight management system shall ensure an efficient search for signs of biological life on Titan.
Delimitations
Delimitations include the exclusion of the following activities due to limited resources or time constraints:
Extensive trajectory optimization and trajectory correction maneuvers
Computational Fluid Dynamics Analysis
CAD Design
Limitations and Assumptions
No limitations and assumptions are specified at this time because the research evaluated multiple principles, methods and technologies presented through the apparatus to define the most efficient characteristics and parameters for the UAS and interplanetary mission design.
List of Acronyms
AGI: Analytical Graphics Inc.
DFD: Direct Fusion Drive
ESA: European Space Agency
GMAT: General Mission Analysis Tool
NASA: National Aeronautics and Space Administration
PCEC: Project Cost Estimating Capability
SPICE: Spacecraft Planet Instrument Camera-matrix Event
STK: Satellite Tool Kit
TSN: Time Sensitive Network
TTN: Time Triggered Network
UAS: Unmanned Aerial Systems
VTOL: Vertical Take-Off and Landing
WBS: Work Breakdown Structure
Literature Review
Titan is the largest moon orbiting Saturn and a solar body that exhibits Earth-like characteristics. In the research study titled: A Titan Mission Using the Direct Fusion
Drive, Gajeri et al. discuss the feasibility and advantages of using a Direct Fusion Drive (DFD) Propulsion system for an unmanned mission from Earth to Titan. The authors highlight the limitations of current combustion-based rocket engines and electrical propulsion systems which includes lower thrust and specific impulse. This leads to significantly high space travel durations that present significant physiological risk to humans. This issue can be mitigated through the use of DFD propulsion systems. They generate significantly higher thrusts and specific impulse which will minimize the overall duration of space flight from Earth to Titan. DFD propulsion systems are essentially a plasma fusion concept which supply the spacecraft with both thrust and electrical energy. The authors confirmed and concluded that an Earth to Titan mission was feasible with DFD systems which optimize flight duration to an interval of 2.6 years with a thrust-coast-thrust profile and less than two years with a continuous thrust profile (Gajeri, Aime, & Kezerashvili, 2021).
Spacecraft propulsion systems with higher capabilities than the common chemical and electrical systems are discussed in the research study titled: Advanced Propulsion for Future Planetary Spacecraft. Garrison presents a thorough overview of spacecraft propulsion system and highlight chemical, laser thermal, solar thermal, electric, fission and fusion based systems. The author discusses the capabilities of a propellant constituted of hydrogen fluoride and nitrogen hydrogen fluoride which include significantly higher specific impulses. It is important to note that this research was completed and published in the early 80’s but its recommendation for a hybrid propulsion system constituted of a chemical propulsion stage and a nuclear electric stage is ideal for an unmanned mission to Titan ( Garrison,1982).
Unmanned Aerial System (UAS) design configurations for planetary exploration are discussed in the research study titled: Evolution of Space Drones for Planetary
Exploration: A Review. Through their study, Hassanalian et al. discuss multiple UAS configuration for space exploration. They highlight the progress made in Space Drones design throughout the years. The authors specify the need to adapt UAS design to each solar body atmospheric characteristics such as density, temperature, and pressure because they will affect the aircraft performance. A methodology for determining the viscosity of Titan’s atmosphere is also presented within this study. It is important to note that the authors recommendation support the use of a flying wing with Vertical Take-Off and Landing capabilities. They also conclude this research by putting an emphasis on a need-based design with requires airframe configuration, material selection, powerplant selection and enabling technologies to be driven by planetary and solar body parameters and atmospheric characteristics to ensure flightworthiness, reliability, and survivability. (Hassanalian, Rice & Abdelkefi, 2018).
A procedure for sizing electrical fixed-wing UAS with VTOL capabilities is discussed in the research study: Comprehensive Preliminary Sizing/Resizing Method for a Fixed Wing-VTOL Electric UAV. Tyan et al. presents a two steps method which consist of first sizing the aircraft solely based on defined design, physical, functional and vehicle performance capabilities requirements. After such, the vehicle is resized based on the characteristics, components, and performance capabilities of the selected propulsion system. The resizing is driven by the additional system mass and its impact on wing loading, thrust to weight ratio and the load factor. In-turn, these parameters drive the necessary update to the wing and tail geometry ( Tyan, Nguyen, Kim, & Lee, 2017).
An analysis and evaluation of the use of a robotic rover to explore Titan is completed in the research study titled: Preliminary Assessment of a Small Robotic Rover for Titan Exploration. Genta & Genta presents characteristics and enabling factors for operating a transportation vehicle on the surface of Titan. Although the study is specific to a wheeled rover, it discusses details and features of Titan’s surface which significantly affects horizontal take-off and landing configurations. The significance of this study with regards to my research is in the authors conclusion that a wheeled transportation system equipped with internal motors can function on the surface of Titan (Genta & Genta, 2011).
The effects of extremely low temperatures on materials are discussed in the research study titled: Properties of Cryogenic and Low Temperature Composite Materials –A Review. Sapi and Butler demonstrate how low temperatures improve the characteristics of composite materials. Colder temperature effect should include lower material Poisson’s ratio with respect to decreasing temperatures because of an increased resin stiffness. But research studies have demonstrated an increased in Poisson ratio with decreasing temperature. Cold temperatures often lead to improved fatigue, strength, modulus, and thermal properties. But the problem that cold temperatures introduce to the use of composite include lower material ductility, lower failure strain, lower fracture toughness and lower resistance to impacts (Sápi & Butler, 2020).
Advanced Materials for future spacecraft applications are discussed in the research study titled: Advanced Materials for Next‐Generation Spacecraft. Levchenko et al. present the need for complete spacecraft autonomy due to the requirements for long duration space flights in environments that displays significant risks like impact with other celestial materials or bodies. The authors assert the need for the development of self-healing materials as a mean to mitigate such risks and improve spacecraft reliability and survivability. The authors also discussed how composites are ideal for developing such capabilities because composites like self-healing polymers already display such intrinsic properties. Levchenko et al. recommend the use of advanced self-healing materials for chassis, antennas, and other critical parts (Levchenko et al., 2018)
Interplanetary missions launch window calculations are discussed in the research study titled: Two-Dimensional Launch Window Method to Search for Launch Opportunities of Interplanetary Missions. Duan and Liu present a two dimensional launch windows calculation method which consist of first defining the launch opportunities range interval and defining sub-intervals with a multi-boundary point search algorithm. After such, the ideal launch window is searched within each defined sub-interval through a random ergodic process. The authors confirm the efficiency of the two dimensional search method in their conclusion. This method reportedly minimize computation when compared to the common pock-chop-plot method. (Duan & Lin, 2020).
The use of synthetic Aperture Radar (SAR) for planetary exploration activities is discussed in the study titled: Space Exploration Synthetic Aperture Radar (SESAR). Rincon et al. presents the characteristics, component, and capabilities of the radar. The higher resolution images generated by the SESAR provide significantly more details including surface and inner-surface details. This radar is ideal for the exploration of solar bodies such as asteroids, and moons like Titan (Rincon et al. 2019)
Optical payloads and enabling technologies are discussed in the textbook titled: Optical Payloads for Space Missions. Qian & Ackleson present a multitude of spaceborne payload and sensors including their usage in previous space exploration missions. The textbook highlights panchromatic sensors, image spectroscopy sensors, imaging spectrometers, multispectral sensors, Fourier transform spectrometers, lidar and active sensors, spectrometers, and radiometers. These technological tools are ideal for the exploration of the surface and inner-surfaces of planets and solar bodies like Titan (Qian & Ackleson, 2016)
The principles governing UAS design are discussed in the textbook titled: Handbook of Unmanned Aerial Vehicles. Valavanis and Vachtsevanos essentially present a comprehensive design guide and best practices for UAS design. This material provides significant details on UAS systems, sub-systems, components, elements, parts, tools and enabling technologies. It specifies a mean for selecting and or designing airframes, powerplants, payload and sensors, launch and recovery methods, emergency procedures, C3 sub-systems, and ground control station. It recommends the use of IR sensors to scan for objects and elements and EO sensors to measure velocities and distances of such objects and elements with respect to the UAS. The authors specify the possibly to incorporate elements detection algorithm with UAS sensors through variables based measurement. Such variable includes temperature or pressure (Valavanis & Vachtsevanos, 2014).
Titan’s physical properties, orbital and body parameters, and atmospheric properties are presented in the textbook titled: Titan: Exploring an Earthlike World. Coustenis and Taylor discuss the similarities between Titan and Earth. They discuss the presence of liquid water, hydrogen and methane, lakes, dunes, and geological features such as volcanoes, mountains, and a rocky body. The variance between atmospheric characteristics is presented in a data table where titan characteristics are expressed in term of a function of Earth’s characteristics (Coustenis & Taylor, 2008)
Methodology
Available Upon Request by contacting me at snahum29@gmail.com prior to an interview.
Results
Available Upon Request by contacting me at snahum29@gmail.com prior to an interview.


Discussion
Available Upon Request by contacting me at snahum29@gmail.com prior to an interview.
Conclusion
Available Upon Request by contacting me at snahum29@gmail.com prior to an interview.
Recommendations
Available Upon Request by contacting me at snahum29@gmail.com prior to an interview.
References
ArianeGroup. (2021, February). Ariane 6 User Manual. https://www.arianespace.com/wp-
content/uploads/2021/03/Mua-6_Issue-2_Revision-0_March-2021.pdf
Atkins, E., Ollero, A., & Tsourdos, A. (2017). Unmanned Aircraft Systems. ProQuest
Ebook Central https://ebookcentral-proquest-com.ezproxy.libproxy.db.erau.edu
Burow, R., Just, D., Adamczyk, A., Jagels, C., Milke, A., Wendt, C., & Kneistler, H. (2019, July).
Thermal Control of the Cryogenic Upper Stage of Ariane 6. 49th International Conference
on Environmental Systems.
Campbell, G., Pope, A., Lazzara, M. A., & Scambos, T. A. (2013, December). The Coldest Place
On Earth:-90 C and Below in East Antarctica from Landsat-8 and other Thermal Sensors.
In AGU Fall Meeting Abstracts (Vol. 2013, pp. C21D-0678).
Coustenis, A., & Taylor, F. W. (2008). Titan: exploring an earthlike world (Vol. 4). World
Scientific.
Curtis, H.D. (2005). Orbital Mechanics for Engineering Students. Elsevier Butterworth
Heinemann
Duan, J., & Liu, Y. (2020). Two-Dimensional Launch Window Method to Search for Launch
Opportunities of Interplanetary Missions. Chinese Journal of Aeronautics, 33(3),
965-977. https://doi.org/10.1016/j.cja.2019.12.010
ESA. (n.d.). What is Electrical Propulsion? Retrieved from https://www.esa.int/Enabling
Support/Space_Engineering_Technology/What_is_Electric_propulsion
Gajeri, M., Aime, P., & Kezerashvili, R. Y. (2021). A Titan Mission Using the Direct Fusion
Drive. Acta Astronautica, 180, 429-438. https://doi.org/10.1016/j.actaastro.2020.12.013
Garrison, P. W. (1982). Advanced Propulsion for Future Planetary Spacecraft. Journal of
Spacecraft and Rockets, 19(6), 534-538.
Genta, G., & Genta, A. (2011). Preliminary Assessment of a Small Robotic Rover for Titan
Exploration. Acta Astronautica, 68(5), 566-56-
https://doi.org/10.1016/j.actaastro.2010.02.016
Guastello, S. J. (2014). Human Factors Engineering and Ergonomics: A Systems Approach
(2nd Ed.) Boca Raton, FL: CRC Press of the Taylor & Francis Group.
Hassanalian, M., Rice, D., & Abdelkefi, A. (2018). Evolution of Space Drones for Planetary
Exploration: A Review. Progress in Aerospace Sciences, 97, 61-105
https://doi.org/10.1016/j.paerosci.2018.01.003
Kemble, S. (2006). Interplanetary mission analysis and design (1st 2006. ed.). Springer Berlin
Heidelberg.
Kwok, S. (2013). Stardust: The cosmic seeds of life (1st 2013. ed.). Springer Berlin Heidelberg.
Levchenko, I., Bazaka, K., Belmonte, T., Keidar, M., & Xu, S. (2018). Advanced Materials for
Next‐Generation Spacecraft. Advanced Materials (Weinheim), 30(50), e1802201-
n/a. https://doi.org/10.1002/adma.201802201
Lorenz, R., & Mitton, J. (2010). Titan unveiled: Saturn's Mysterious Moon Explored. Princeton
University Press.
Qian, S., & Ackleson, S. G. (2016). Optical Payloads for Space Missions. Wiley.
Rincon, R., Carter, L., Lu, D., Du Toit, C., Perrine, M., Hollibaugh-Baker, D. M., & Neish, C. D.
(2019, July). Space Exploration Synthetic Aperture Radar (SESAR). In IGARSS 2019-
2019 IEEE International Geoscience and Remote Sensing Symposium (pp. 8320-8323).
IEEE.
Sápi, Z., & Butler, R. (2020). Properties of Cryogenic and Low Temperature Composite
Materials–A Review. Cryogenics, 103190.
Schroder, K.P., Smith, R. C. (2008, March 13). Distant Future of the Sun and Earth Revisited,
Monthly Notices of the Royal Astronomical Society, Volume 386, Issue 1, May 2008,
Pages 155–163, https://doi.org/10.1111/j.1365-2966.2008.13022.x
Tyan, M., Nguyen, N. V., Kim, S., & Lee, J. (2017). Comprehensive Preliminary Sizing/
Resizing Method for a Fixed Wing – VTOL Electric UAV. Aerospace Science and
Technology, 71, 30-41. https://doi.org/10.1016/j.ast.2017.09.008
Uhlig, T., Sellmaier, F., & Schmidhuber, M. (Eds.). (2015). Spacecraft Operations. Springer
Vienna.
Valavanis K.P., Vachtsevanos G.J. (2015). Handbook of Unmanned Aerial Vehicles. Dordrecht,
Netherlands: Springer Publishing Company.
コメント