Analysis of The Development and Usage of Nuclear Pulse Propulsion Systems for Spacecraft
- Setondji V. Nahum
- Nov 13, 2020
- 10 min read
Course: Application in Space: Defense, Commercial and Exploration
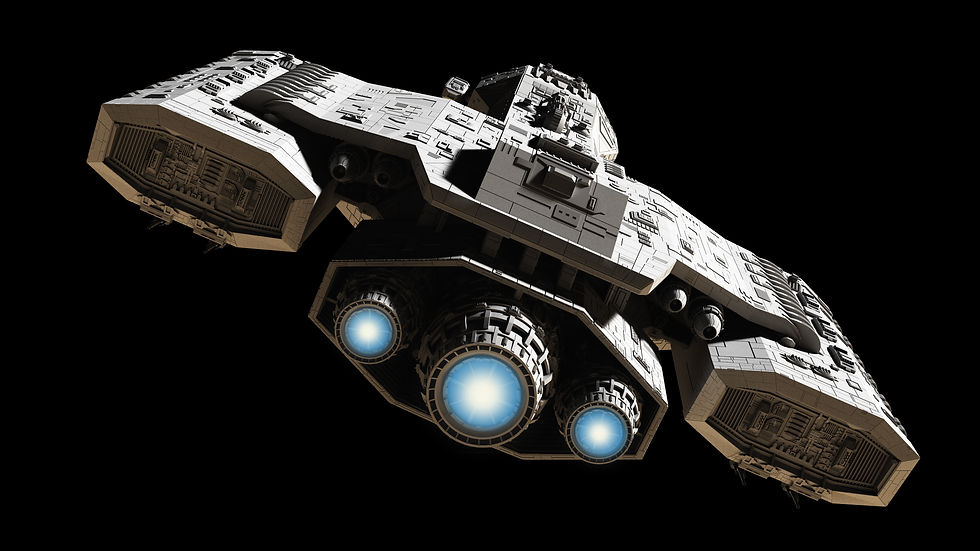
Introduction
Velocity is the rate of change of position with respect to a defined time interval and a frame of reference. It is a critical performance characteristic that is used to select or design the ideal type of spacecraft for an exploration mission. Spacecraft engines generate the thrust forces that accelerate the spacecraft and cause the rapid displacement which is often characterized as the speed of the spacecraft. It is essential to missions from Earth to other interplanetary bodies or extra-solar planets since the distance between planet Earth and these planets are significantly high. The need for speed has led engineers to research and develop multiple type of spacecraft propulsion systems. Although the most common systems used are chemical propulsion systems, significant advances have been made in studies of electrical propulsion systems and fuel cell engines. An intriguing solution to the need for significantly faster space travel is the development and usage of nuclear propulsion systems including nuclear pulse propulsion (NPP) systems.
The achievement of humans’ objectives such as manned mission to other planetary bodies and extra-solar planets, and the development of human habitat across the solar system will require rocket engines with significantly higher performance characteristics and capabilities. Chemical propulsion systems are limited and characterized by low specific impulse and specific power (Mattingly, 2006). Although the development of electrical propulsion systems provided a mean for higher specific impulse, these types of systems do not generate sufficient thrust. Mankind must find faster ways to travel across the solar system and the cosmos. Long duration space flight is a significant hurdle to human space exploration efforts due to its detrimental impact on human physiology (Niehoff et al., 2016). Spacecraft equipped with external pulse plasma propulsion systems are capable of revolutionizing space exploration by significantly minimizing space flight durations due to their higher performance characteristics and the capability to generate significantly higher specific impulse and specific power (Schmidt, Bonometti & Irvine 2002). What are the advantages and disadvantages of the development and usage of nuclear pulse propulsion systems for manned and unmanned spacecraft?
Background and Analysis
1. Methodology
Researching the advantages and disadvantages of the development and usage of nuclear pulse propulsion systems was completed through documentation analysis. The first step was to research relevant textbooks that discuss fundamentals elements of propulsion, rocket engines and nuclear propulsion systems. The second step was to select a legitimate source of documented research. The third step was to define the criteria for materials to be selected. Such criteria were performance characteristics, enabling technologies, environmental impacts, and human factors. Per the specified criteria, table 1 displays the textbook and research studies selected.
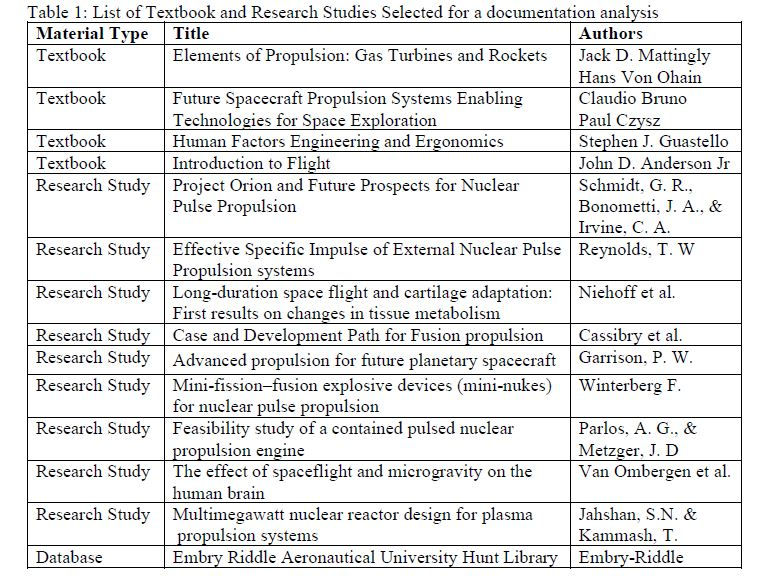
2. Fundamental elements of rocket propulsion
An analysis of the performance characteristics of nuclear pulse propulsion systems requires an understanding of the fundamentals elements of rocket propulsion such as thrust, specific impulse and propulsive efficiency.
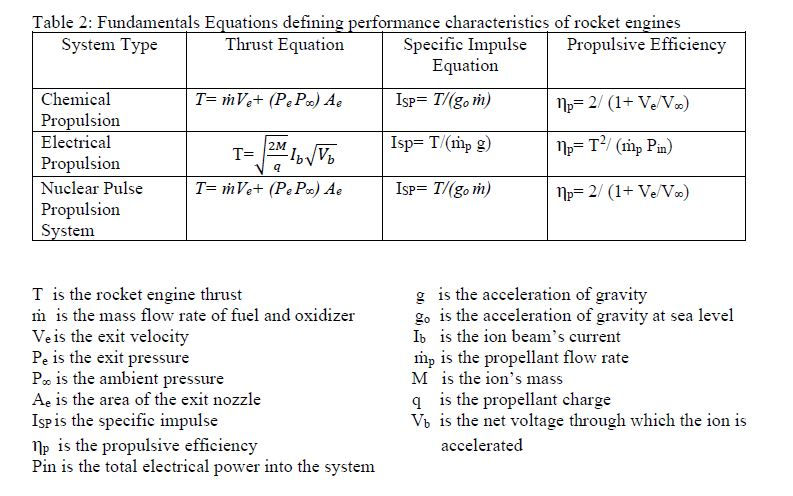
It is important to note the similarity in the mathematical principle for determining thrust and specific impulse for chemical and nuclear pulse propulsion systems. The fundamental difference in these two systems is the method or mechanism used to accelerate the propellant mixture and the resulting effect on the change in initial pressure and exit pressure after the detonation in the case of NPP systems (Parlos & Metzger, 1994).
3. Overview and Limitations of Chemical and Electrical Propulsion Systems
Chemical systems produce thrust by combusting fuel and oxidizers to create a high-temperature, high-pressure mixture of combustion products that is pushed through a rocket nozzle (Anderson, 2016). Chemical propulsion systems are characterized by the weight of fuel and propellant which often represent approximately 80% of the spacecraft systems weight (Mattingly, 2006). Their limitation includes low specific impulses. In contrast to violent chemical reactions, electrical systems produce thrust by using electrical power to accelerate low-molecular weight gazes through electrical and or magnetic means (ESA, n.d.). Although they generate higher specific impulses, they are limited by low thrusts production.
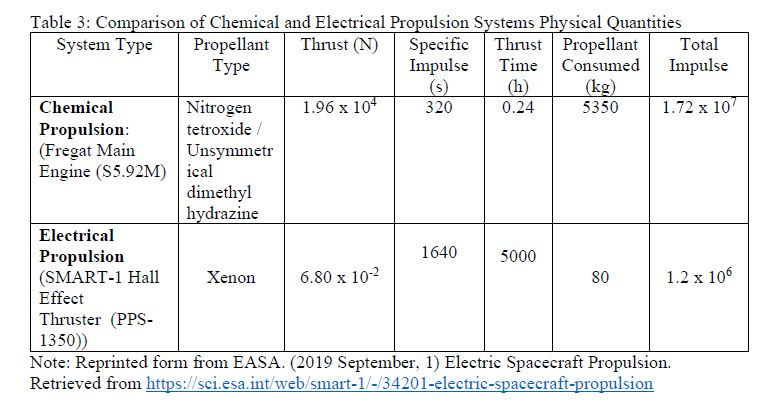
4. Nuclear Pulse Propulsion Systems
a. Propulsive Mechanism
Nuclear Pulse Propulsion (NPP) systems essentially consist of using explosive pulses to propel the spacecraft. There are two fundamental concepts for NPP: external pulse propulsion and internal pulse propulsion. External pulse propulsion consists of ejecting a pulse unit behind the spacecraft and using the pulse energy to push the spacecraft forward. The pulse unit is composed of a mixture of propellant and materials that undergo fission or fusion. When the pulse is ejected, the propellant mass expands and reach the pusher plate of the spacecraft. Through momentum conservation, the spacecraft gains momentum, and its specific impulse increases (Reynolds, 1973).
The mechanism of internal pulse propulsion is similar to the chemical combustion concept. As opposed to a chemical combustion, a nuclear reaction or explosion occurred in a pressure vessel and the heated propellant is pushed through a nozzle (Schmidt, Bonometti & Irvine 2002).
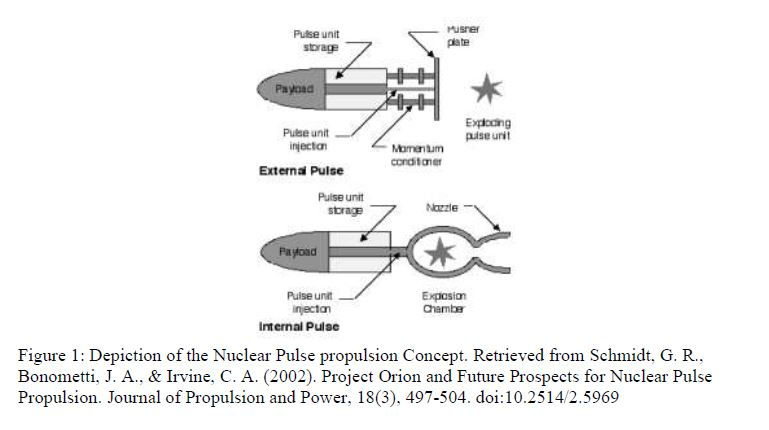
b. NPP Performance characteristics
Figure 2 display the mass flow rate of the propellant mixture when the pressure in the containment vessel is less than the critical pressure and the mixture is released.
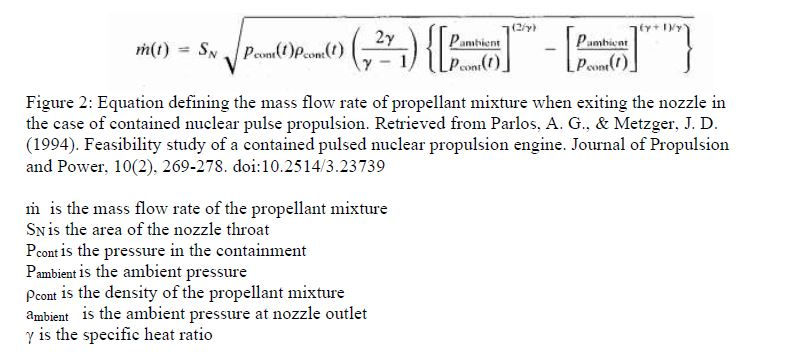
Figure 3 displays the derived rocket thrust and specific impulse equations.
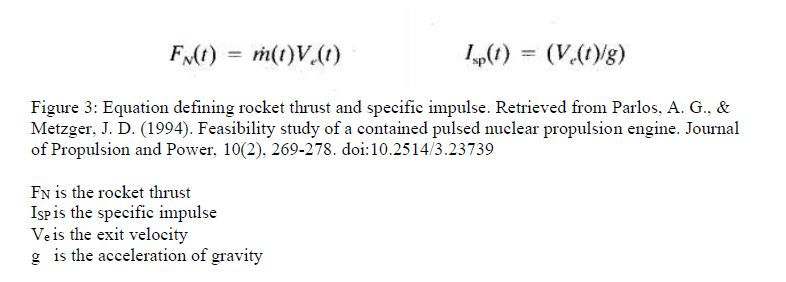
NPP systems have generally demonstrated the capability of generating specific impulse ISP in the range of 10000 sec and thrust of 1000 KN (Reynolds, 1973). NPP systems have the capability of generating specific impulse (Isp) above 50000 sec through the use of magnetic shielding (Schmidt, Bonometti & Irvine 2002).
c. Design Configuration and Characteristics
Figure 4 describes the first spacecraft configuration defined through the Orion Project and NASA’s configuration of the Orion spacecraft.

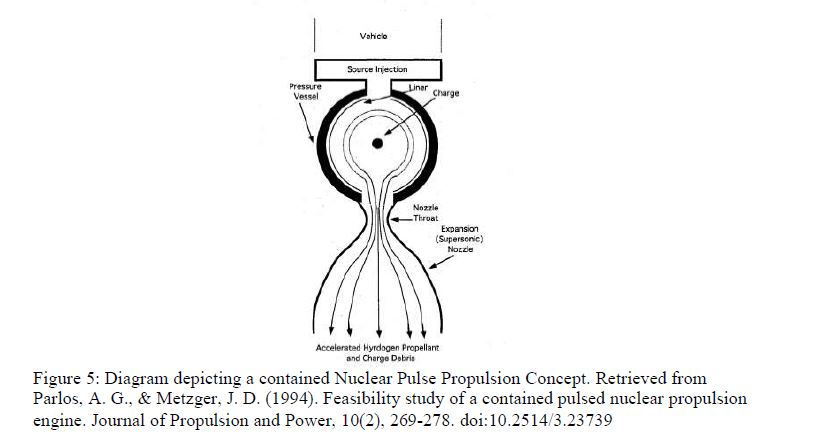
5. Enabling Technologies for the design of Nuclear Pulse Propulsion Engines
The nuclear pulse propulsion concept was pioneered by the Orion project in the 1960’s. It led to a multitude of derived concepts and the development of remarkable machineries. Throughout the years significant progress have been made in the field of technology and multiple technological innovation can be adapted to the Orion spacecraft and the concept of nuclear pulse propulsion. Jahshan and Kammash of the Department of Nuclear and Radiological Sciences concluded in their research study of multimegawatt nuclear reactor design for plasma propulsion systems that an increased radial reflector thickness for lower power (under 10-MWe) reactors is an advantage in mass and volume savings at no cost in design and safety capabilities (Jahshan & & Kammash, 2005). This reactor concept is ideal for internal pulse propulsion systems and it can be optimized and adapted for use on both internal and external NPP spacecraft.
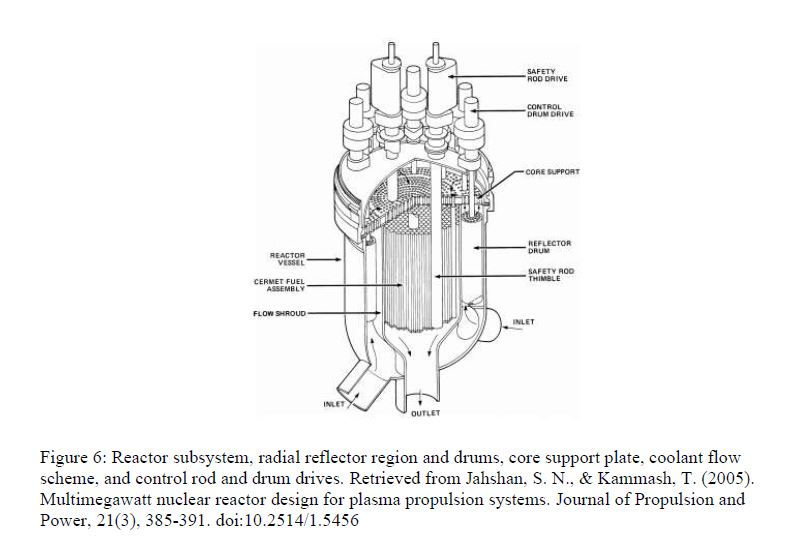
Winterberg presents the concept of maximizing nuclear energy use for NPP systems through the use of mini-nukes. In the case of external pulse propulsion and since they are much smaller, the explosion can occur in close proximity of the pusher plate in the focus of a parabolic reflector. In the case of internal pulse propulsion, the explosion can occur in a pressure vessel or combustion chamber.
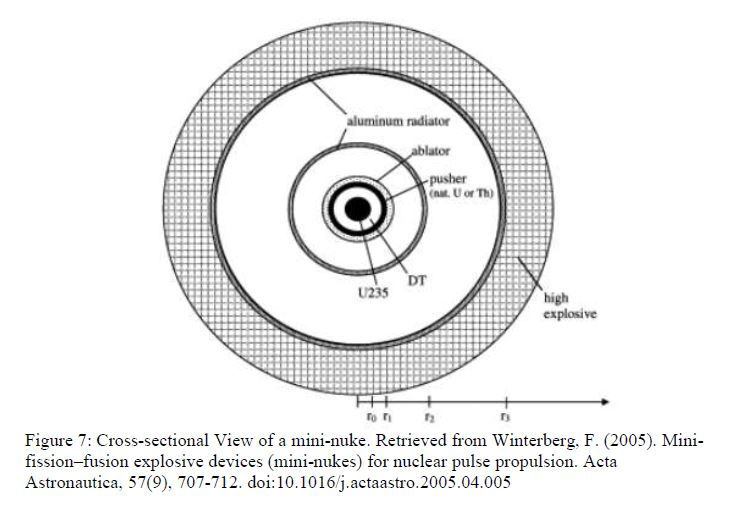
Other enabling technologies shall include shock absorbing technologies to mitigate the effects of sudden spacecraft acceleration per pulse on the spacecraft passengers, the spacecraft structure, subsystems, and components. Advancements made in the development of composite materials for spacecraft structures, spacecraft health monitoring technologies, guidance and navigation technologies, safety systems, human factors and human support sub-systems and technologies.
Evaluation and Interpretation
An evaluation of the development and usage of nuclear pulse propulsion systems require a systems approach. Significant factors shall include elements such as performance characteristics, design configurations, environmental emissions, and pollution, enabling technologies, global nuclear regulations and laws, support infrastructures, life-cycle costs, and human factors.
1. Comparison of Nuclear Pulse to Chemical and Electrical Propulsion Systems
Table 4 displays a comparison of engine performance characteristics between nuclear pulse, chemical and electrical propulsion systems.

The data presented in table 4 demonstrate the superiority of nuclear pulse propulsion systems. Contrary to chemical and electrical propulsion systems, NPP systems are not limited by low specific impulse or low thrusts. In fact, they generate significantly higher thrust and specific impulse. This optimizes program and mission capabilities. It facilitates and fosters the development of both manned and unmanned mission to extra solar planets and distant planets such as Jupiter, Neptune, and Uranus.
2. Advantages of Nuclear Pulse Propulsion Systems
Nuclear pulse propulsion systems substantially minimize the duration of space travel and space missions. With specific impulse in the range of 10000s to 50000 s, Jupiter can be reached from earth within 200 days or less and Mars can be reached within 28 days or less. This is supported by Cassibry et al. who reported that with specific impulse in the range of 50000s, Jupiter is reachable from earth in 42 days (Cassibry et al., 2015). NPP systems enhance program and mission capabilities and significantly accelerate the rate of space exploration by humans.
A significant minimization of flight duration limits the effect of gravity on human physiology. In fact, prolonged exposure to microgravity has a detrimental impact on humans. It leads to a degradation of bone cartilage, a loss of bone density and muscle atrophy (Niehoff et al., 2016). Along with microgravity, isolation, immobilization, and confinement also have a harmful impact on the human brain. They contribute to a deregulation of humans’ circadian rhythm, and they are the cause of significant psychological problems, cephalic fluid shifts, neurovestibular problems and cognitive alteration ( Van Ombergen et al., 2017). Although the impact of these conditions on human physiology is still being researched, it is rational to conclude that a mitigation or minimization of exposure to such conditions is critical to space exploration. One of the solutions is to achieve faster travel and limit the amount of time spent in microgravity, confined and immobile conditions. Nuclear pulse propulsion systems enable such solutions with their capabilities for significantly higher specific impulse and thrust. As opposed to being under detrimental conditions throughout years of travel, astronauts may only have to endure such conditions for a few weeks or months. It is a tremendous improvement which may also allow for a focus on the development of other technologies such as life support systems during flight and life support habitats on other planets.
3. Disadvantages of Nuclear Pulse Propulsion Systems
Nuclear Pulse Propulsion systems research development efforts were abruptly halted by the Limited Test Ban Treaty of 1963. Researchers and Scientists were on the midst of developing engineering solutions to issues related to NPP usage for spacecraft and any environmental challenge or problems faced by NPP usage. Robust risks management plans were being developed with the main objective of mitigating environmental issues (Schmidt, Bonometti & Irvine 2002). When it comes to disadvantages, one of the most significant issues is humans’ uneducated perceptions and uninformed reasonings with regards to potential environmental impacts. An attempt to develop and use NPP systems for spacecraft may create significant bureaucratic and political issues as well as a public outrage which may become detrimental to the program.
The determination of the actual environmental impact of NPP development and usage for spacecraft depends on defined program criteria and specifications. The most complex environmental scenario is a ground launch using the NPP engine. This presents significant disadvantage as it will require the development of unique infrastructures and technologies aimed at containing radiations and diffusion into Earth’s atmosphere. A potential solution is a ground launch through the use of a chemical propulsion system or rocket boosters followed by an activation of the NPP systems once the spacecraft has reached earth’s orbit or a safe location in space. In fact, NPP systems can safely be ignited or launched in space or in an earth orbit where no environmental risk is presented to earth or the space environment itself. Solar winds and cosmic rays constantly generate radiations of significantly higher magnitude into space than any radiation magnitude that can be generated by NPP systems. In fact, Project Orion engineers determined that an extensive NPP spacecraft flight program would contribute to no more than an additional 1% to the atmospheric contamination generated by open-air nuclear weapons tests (Schmidt, Bonometti & Irvine 2002).
Other disadvantages pertains to facing the challenge of establishing a new spacecraft program, the need to develop and or improve solutions to technical and product integration challenges such as optimizing pulse unit performance, converting pulse unit energy into vehicle momentum, and improving vehicle design and technologies (Schmidt, Bonometti & Irvine 2002). Program development cost and spacecraft and nuclear technology lifecycle cost may also be a disadvantage because it is still an unknown parameter. The need to integrate the most advanced technologies and enable interoperability between the spacecraft sub-systems and between the spacecraft, human operators and ground-based flight management systems may lead to a significant increase in program development cost.
Conclusion
The achievement of faster space travel to other solar system planets, extra-solar planets and cosmic bodies will require rocket engines with significantly higher performance characteristics and capabilities. Contrary to chemical and electrical propulsion systems, Nuclear Pulse Propulsion systems are not limited by low specific impulse or low thrusts. In fact, they generate significantly higher thrust and specific impulse. NPP systems can reportedly generate thrust in the order of 1000 x 103 KN and specific impulse in the range of 10000s to 50000s. This indicate that spacecraft powered by NPP systems can reach a distant planet like Jupiter within approximately 200 days to 42 days. It enhances program and mission capabilities and significantly accelerate the rate of space exploration by humans. Given the detrimental impact of microgravity, isolation, confinement, and immobility on humans’ physiology, NPP systems offers a solution for limiting the time spent under such arduous conditions. The most significant disadvantage of NPP systems is the risk of emitting radiations, diffusions, and toxic pollutants into Earth’s atmosphere through a ground launch of the spacecraft. But a potential solution to this issue is a ground launch through the use of a chemical propulsion system or rocket boosters followed by an activation of the NPP systems once the spacecraft has reached earth’s orbit or a safe location in space.
References
Mattingly J.D. (2006). Elements of Propulsion: Gas Turbine and Rockets. Reston, Virginia:
American Institute of Aeronautics and Astronautics, Inc.
Niehoff, A., Brüggemann, G. , Zaucke, F., Eckstein, F., Bloch, W., Mündermann, A., . . .
Liphardt, A. (2016). Long-Duration Space Flight and Cartilage Adaptation: First Results on
Changes in Tissue Metabolism. Osteoarthritis and Cartilage, 24, S144-S145.
doi:10.1016/j.joca.2016.01.282
Schmidt, G. R., Bonometti, J. A., & Irvine, C. A. (2002). Project Orion and Future Prospects for
Nuclear Pulse Propulsion. Journal of Propulsion and Power, 18(3), 497-504.
doi:10.2514/2.5969
Bruno, C., & Czysz, P. (2009). Future Spacecraft Propulsion Systems Enabling Technologies
for Space Exploration (2nd ed.). Springer Berlin Heidelberg. https://doi.org/10.1007/978-3-
540-88814-7
Garrison, P. W. (1982). Advanced propulsion for future planetary spacecraft. Journal of
Spacecraft and Rockets, 19(6), 534-538. doi:10.2514/3.62297
Parlos, A. G., & Metzger, J. D. (1994). Feasibility Study of a Contained Pulsed Nuclear
Propulsion engine. Journal of Propulsion and Power, 10(2), 269-278. doi:10.2514/3.23739
EASA. (2019 September, 1) Electric Spacecraft Propulsion. Retrieved from
Reynolds, T. W. (1973). Effective Specific Impulse of External Nuclear Pulse Propulsion
Systems. Journal of Spacecraft and Rockets, 10(10), 629-630. doi:10.2514/3.61941
Jahshan, S. N., & Kammash, T. (2005). Multimegawatt Nuclear Reactor Design for Plasma
Propulsion Systems. Journal of Propulsion and Power, 21(3), 385-391.
doi:10.2514/1.5456
Winterberg, F. (2005). Mini-Fission–Fusion Explosive Devices (mini-nukes) for Nuclear Pulse
Propulsion. Acta Astronautica, 57(9), 707-712. doi:10.1016/j.actaastro.2005.04.005
Cassibry, J., Cortez, R., Stanic, M., Watts, A., Seidler, W., Adams, R., . . . Fabisinski, L. (2015).
Case and Development Path for Fusion Propulsion. Journal of Spacecraft and
Rockets, 52(2), 595-612. doi:10.2514/1.A32782
Van Ombergen, A., Demertzi, A., Tomilovskaya, E., Jeurissen, B., Sijbers, J., Kozlovskaya, I.
B., . . . Wuyts, F. L. (2017). The Effect of Spaceflight and Microgravity on the Human
Brain. Journal of Neurology, 264(S1), 18-22. doi:10.1007/s00415-017-8427-x
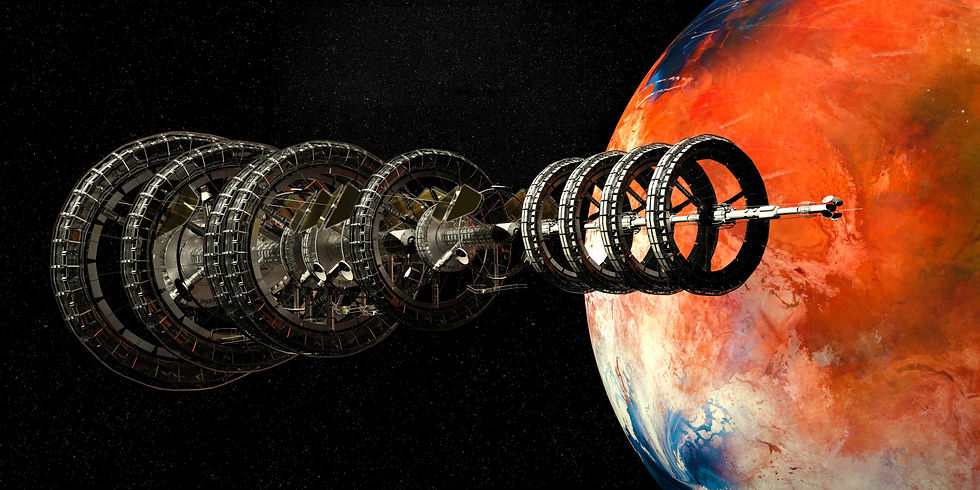
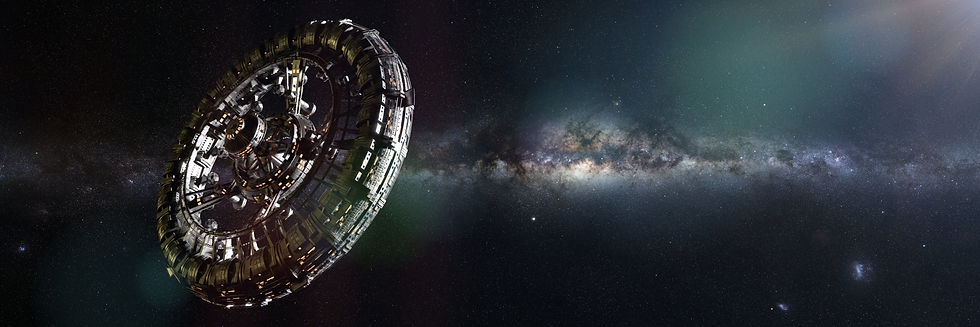
Commentaires